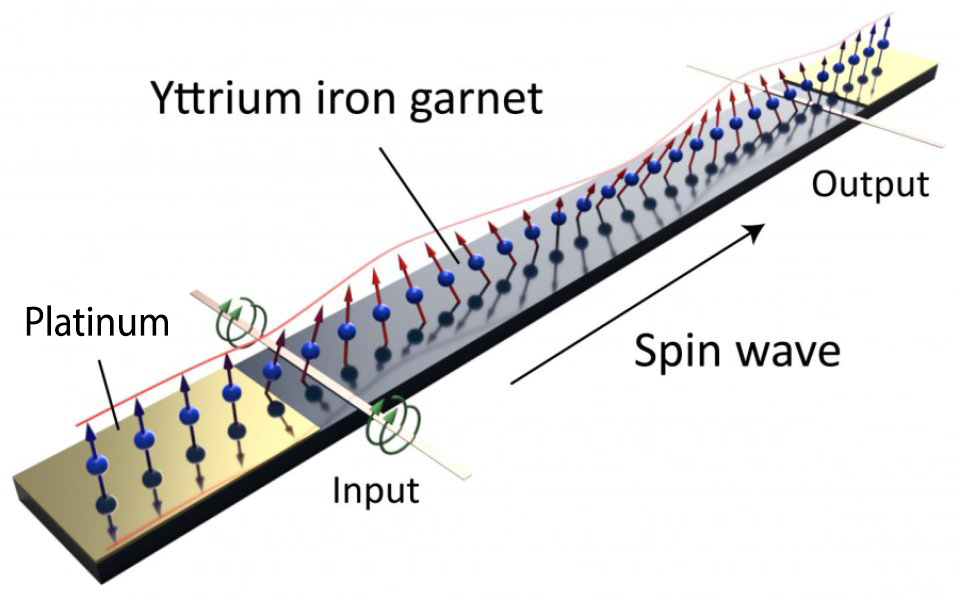
As we know from experience with our mobile electronics, heavy usage heats things up. As devices consume energy for work, a non-negligible amount of energy is wasted as heat. Not only is this unideal from a conservation standpoint, but it is generally not good for the electronics either. This is especially a problem as device size shrinks as there is more power being dissipated in a smaller space. Research performed in the Krivorotov lab here at UCI, lead by Dr. Chris Safranski, has developed a method to use heat dissipated in electronics to generate microwaves.
The Krivorotov group focuses on a field called spintronics, the use of the spin of the electron as the carrier of information in electronic devices instead of traditional electric charge. This is one of the main applications of magnetism today. When one discusses magnetism, the spin of an electron is commonplace. Spin is an inherent quantum characteristic of a particle, like its charge. The name comes from its analogy with classical angular momentum. The spin and orbits of electrons in a solid generally point in random directions, which average to zero over a macroscopic volume. However, in magnetic materials, these spins and orbits can align in certain preferential directions in an external magnetic field, inducing a magnetization and a magnetic field of their own.
The Krivorotov group has fabricated a device to drive excitations in a magnetic material using a normal metal. The excitations generated are spin waves. An example of a spin wave can be imagined if considering a solid where the spins of all electrons are pointing up. Say an electron on one side of the material’s spin is perturbed off vertical a little. This perturbation can be transferred to the electron next to it and it returns to its original state, creating a domino effect. As this perturbation travels down the line, one has a spin wave.
Electrons in a normal metal are relatively free within the confinement of the solid and do not interact much, whereas electron-electron interactions are crucial to magnetism. At the interface a normal metal and a ferromagnet (a material which retains its magnetization), an electron’s spin in the normal metal will interact with the aligned spins in the ferromagnet. This can change the angular momentum of the spin through an effect called Spin Transfer Torque. Through this transfer of angular momentum, the system tries to come to some equilibrium; the system will undergo magnetization auto-oscillations. This is the origin of how the Krivorotov group can drive spin wave excitations.
To drive these excitations, one can realize that thermal gradients induce currents through diffusion. As a solid heats up, the electron energies shift. Electrons on the hot side will diffuse to the colder section at a lower energy, approaching some equilibrium distribution (the Seebeck effect). This thermal gradient induces a current. As electrons in the normal metal pass by the interface with the ferromagnet, the excitations described above will be induced and magnetization auto-oscillations will occur in the ferromagnet. With these auto-oscillations, one can use magnetoresistive effects to obtain a usable signal from Ohm’s law δVac = IDC δRac. These auto-oscillations occur at GHz frequencies, radiating microwaves. The oscillation frequency depends upon both the sample material and the geometry.

The Krivorotov lab designed a device that has demonstrated this principle, shown above in figure 1. Placing a magnetic insulator, Yttrium-iron-garnet (YIG), under a platinum wire (a “normal” metal), one can heat this system by passing current through the metal. In the presence of an external magnetic field, microwave signals are observed. The signals emitted from these devices were too low to be measured by traditional techniques, so they had to develop their own, modulating the applied magnetic field. Their new technique increased the signal to noise by two orders of magnitude, as shown in figure 2.

Regarding the work, Safranski said, “probably my favorite part of the project was finally getting the first signal from the device. The materials involved made fabrication of these devices difficult and required finding new nanofabrication techniques. On top of that the expected signal strength was low, so we had to develop a new measurement technique to be able to get a measurable signal.” Some of the tools and training needed for this type of project include clean room and nanofabrication, microwave electronics, and magnetization measurement techniques, as well as finite element computer simulations. While the output power is not enough to cool the devices it is certainly an intriguing way to recover useful work from otherwise wasted energy. Current work is aimed to increase the output power.
Chris Safranski’s research interests lie in magnetic materials and their potential applications towards nanoscale devices. Chris graduated from Ilya Krivorotov’s group in 2018 and is now a postdoctoral researcher for IBM working for the MRAM group at the T.J. Watson Research Center in New York. The publication can be found here: “Spin caloritronic nano-oscillator,” Nature Communications. 8, 117 (2017).
Post by Mackenzie Turvey, graduate student working on his PhD in Condensed Matter Experiment at UCI.